AstroCeNT — the ultra-modern Particle Astrophysics Science and Technology Centre of the Polish Academy of Sciences — was established in Warsaw in 2018. Currently, under the leadership of Prof. Leszek Roszkowski the Centre implements its research program, attracting scientists from all over the world. Dr. Marcin Kuźniak joined the team in 2019 as the leader of the group that is working on the improvement of silicon photomultipliers — technology extremely important for both science and industry. Marcin told us about his path to astrophysics, 10-years’ experience in Canada and return to Poland, as well as about the projects developed by AstroCeNT.
– Astrophysics as a science has been around for many years, but it still sounds very intriguing to the common person. So, the first question — how does one become an astrophysicist?
– My path to astrophysics began a long time ago with my interest in physics. As a child, I was very curious about how it all worked. I started reading popular science books, also a lot of science fiction; I had a lot of thoughts connected with it — partly scientific, partly philosophical… As a result, I decided to study physics, although it was not the only field of study I was considering. At the university I got involved in research projects of my supervisor’s experimental group, and then everything started: travelling abroad, cooperation with foreign groups. After all, since I left Poland in 2005, apart from short visits, I only came back a year ago.
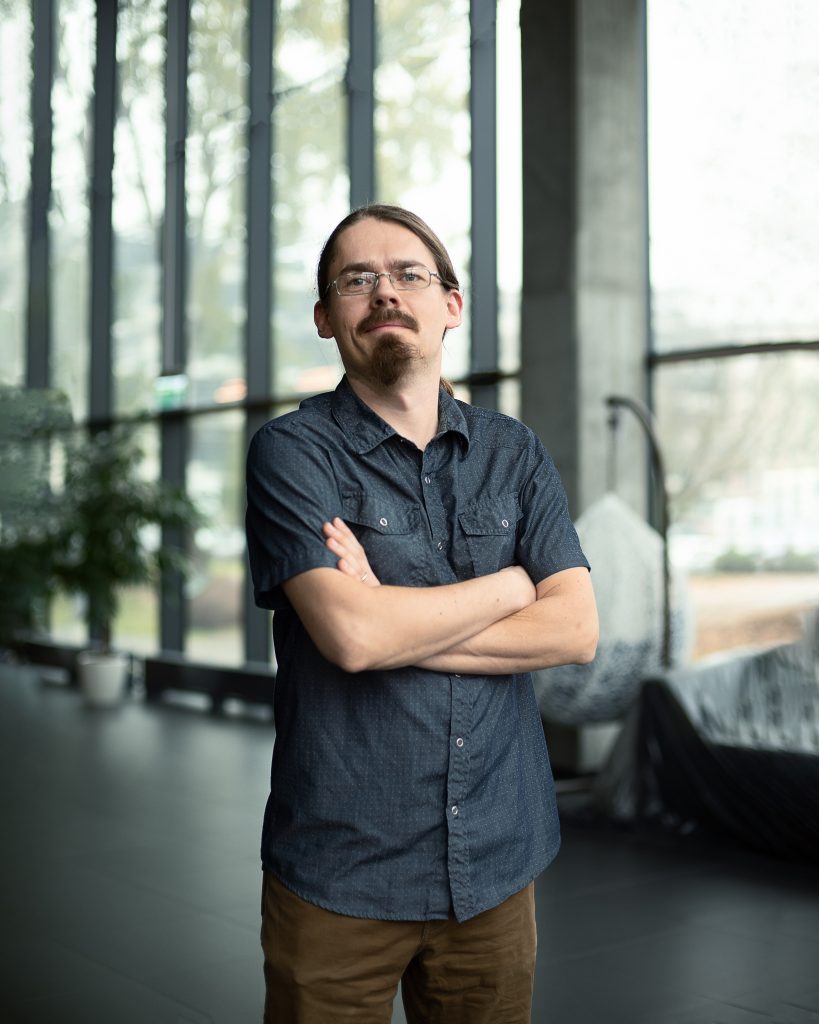
– But did you go in the direction of astrophysics by accident?
– My doctoral thesis was related to a different topic. It was about pure particle physics and nuclear physics — an extremely precise measurement of the electric dipole moment of a neutron, which also indirectly has astrophysical implications. It is a long-term project in which I participated in the initial phase. The actual experiment started much later, but at that early stage we managed to find a way to use the developed equipment for the experiment that put limitations on one of the possible scenarios explaining the mystery of dark matter. We made a measurement, which became a part of my doctoral dissertation, and it was precisely the moment when I came to this subject more closely.
In 2009, while looking for a postdoctoral position, I went in this scientific direction. Also I followed my future wife, who was already in Canada. As a result, I ended up in Kingston and that’s how my adventure with dark matter began.
– …An adventure that continues today?
– Yes, I am currently involved in experiments looking for particles of so-called dark matter that we believe is filling the universe. We know about the existence of dark matter from astronomical observations and astrophysics. Analyses of the speed at which stars in galaxies, or galaxies in galaxy clusters move, clearly show that ordinary matter (which emits or absorbs light) is only about one fifth the mass of all matter in the universe. Galaxies and other larger structures of ordinary matter are surrounded by a “cloud” of dark matter that has mass and gravitationally attracts, but is invisible because it does not glow or cast a shadow. We suspect that dark matter may be created by elementary particles with special properties that have not been registered yet, although predicted by some theories, and we are trying to find direct evidence of their existence.
– Tell a few words about the university in Kingston where you worked.
– Queen’s University of Kingston has a rich tradition in the dark matter field. Its scientific group was one of the most pivotal in the SNO (Sudbury Neutrino Observatory) experiment, which was a great success. It has solved the problem of solar neutrinos — a puzzling discrepancy between predictions and measurements of the amount of these volatile particles reaching us from the interior of the sun. In 2015, Art McDonald, leader of the SNO experiment, won the Nobel Prize for discovering neutrino oscillations, and in 2016, the entire SNO team, along with other neutrino experiments, received the prestigious Breakthrough Prize.
A laboratory was created for the SNO detector — 2 km underground, in the nickel mine in Sudbury, which is still operating today. The underground location is necessary to shield the detector from cosmic rays, which would completely jam the signal in the detector, both from neutrinos and dark matter. For the same reason, natural radioactivity is a problem, which is why detectors are usually made of specially selected ultra-pure materials.
Later, the laboratory was expanded, and today there are several experiments there, which deal with either neutrinos or dark matter — a large part of them grew out of SNO, use similar technologies and are operated or led by former SNO participants. At Queen’s there are people who started this avalanche. Students of that time, who are already professors, and former professors, who, although mostly retired, still actively participate in the life of the department: they regularly attend seminars, coffee meetings, observe the work of young physicists, advise, review papers and internal reports, and act as mentors.
– What was your job at Kingston about?
– I worked in the DEAP experiment group, also located at SNOLAB, in which we are searching for dark matter with a liquid argon detector. My immediate supervisor was Mark Boulay, who is also the head of the entire experiment. Later our group partially moved to Carleton University in Ottawa, but it was still the same project. The stage in Kingston focused on research and development and construction of the detector, in Ottawa we dealt with the analysis of data flowing from it.
– What are your personal impressions of this experience?
– Apart from the challenges of the Canadian winter, Kingston and Ottawa are very friendly places to work and live, and unfortunately, we left a lot of friends there. These were certainly very productive years. There is so much work to do that I have less and less time to admire the cosmic context of our research on a daily basis, or the quite unique specifics of it (smiles), but it can be really fun. The very awareness of working on such a project gives a lot of inspiration.
What the SNO team did in the 80’s and 90’s building the detector is unbelievable. It was connected first with the construction of an ultrapure laboratory in an active and operating mine, i.e. conditions that were far from cleanliness, and then with the detector itself; working hand in hand with miners in very difficult conditions. The project encountered many difficulties already at the stage of raising funds for implementation. There were also at least a few moments where it seemed that technical complications would put it to an end. But the team managed to fix the issues every time. People who did all that paved the way for the younger generation of physicists. Our challenges in working on DEAP, compared to what they faced, seemed small. So, instead of feeling sorry for ourselves, we just looked for solutions.
– Did you leave this project after moving to Warsaw?
– No, I didn’t. A feature of modern large experiments, including those looking for dark matter, is that they are multi-stage. First, we develop or test the necessary technologies, then we build the detector. It works for several years, reaching a certain maximum sensitivity, usually limited by the background from natural radioactivity.
To move the search forward, there is no other option than building a new detector, bigger and better. Having a larger amount of mass in the detector, we obtain sensitivity to weaker and less frequently interacting particles. Of course, the problem itself is to ensure the purity of this mass from radioactive isotopes. With each subsequent level, this construction becomes more expensive and more difficult. By building detectors of increasing sensitivity and scale, we often come across new problems that we had no idea about and which we learn to solve on the way to the goal.
– So, the DEAP experiment is still going on, but you are starting to build another one in parallel?
– Yes, the Canadian team I have worked with is currently working with groups around the world to develop a global agenda for further research. Other Polish groups also participate, in particular a group from the Jagiellonian University. In 2018, a global cooperation was established to build next-generation liquid-argon detectors. First 10 times larger — and this is already happening in Italy (DarkSide-20k experiment). And in the next decade, it is planned to build a detector 100 times larger — most likely again in Canada, in SNOLAB.
Recently, together with four other institutions from this collaboration (Italian INFN and GSSI, French CNRS and German TUM), we received funding from the European Commission for closer cooperation and for the development of new joint lines of action in the context of gravitational waves and neutrino physics. This project, DarkWave, has just started. Therefore, I have to divide my time between working on the previous project and participating in new initiatives.
– Let’s go back to Warsaw. Tell us about how you came to AstroCeNT.
I heard about AstroCeNT at the DarkSide collaboration meeting (such meetings are held several times a year). Prof. Leszek Roszkowski announced that AstroCeNT has just been created and candidates for group leaders are being sought. At that time, I was looking for a permanent position, i.e. a place where I could create my own group and gain scientific independence, so I decided to apply. AstroCeNT was very attractive for at least several reasons.
First, the International Research Agendas program, implemented by Foundation for Polish Science and financed by the European Regional Development Fund, is still new. At least in our field, nothing like this has ever happened in Poland. In Canada and the United States, open competitions for professorships are the norm, and winners automatically receive substantial funding to hire people, buy equipment, equip laboratories and to make research. The conditions at AstroCeNT were very competitive in this respect, even on a global level. I didn’t think that I would see such an opportunity in Poland.
Second, the center of gravity for the search for dark matter is now shifting to Europe, as the next experiment, DarkSide-20k, will be in Italy. And for a long time I had a clear idea for my unique contribution to this project and was looking for a place where I could implement it. It all fit together quite well.
– And the possibility of returning to Poland in itself? Was it one of the reasons?
Yes, I decided that this is the last moment to come back and see if it is possible to continue a career in my field in our country. With full awareness that it will be an adventure, but also that it involves some risks, and among them the fact that there was no such program before.
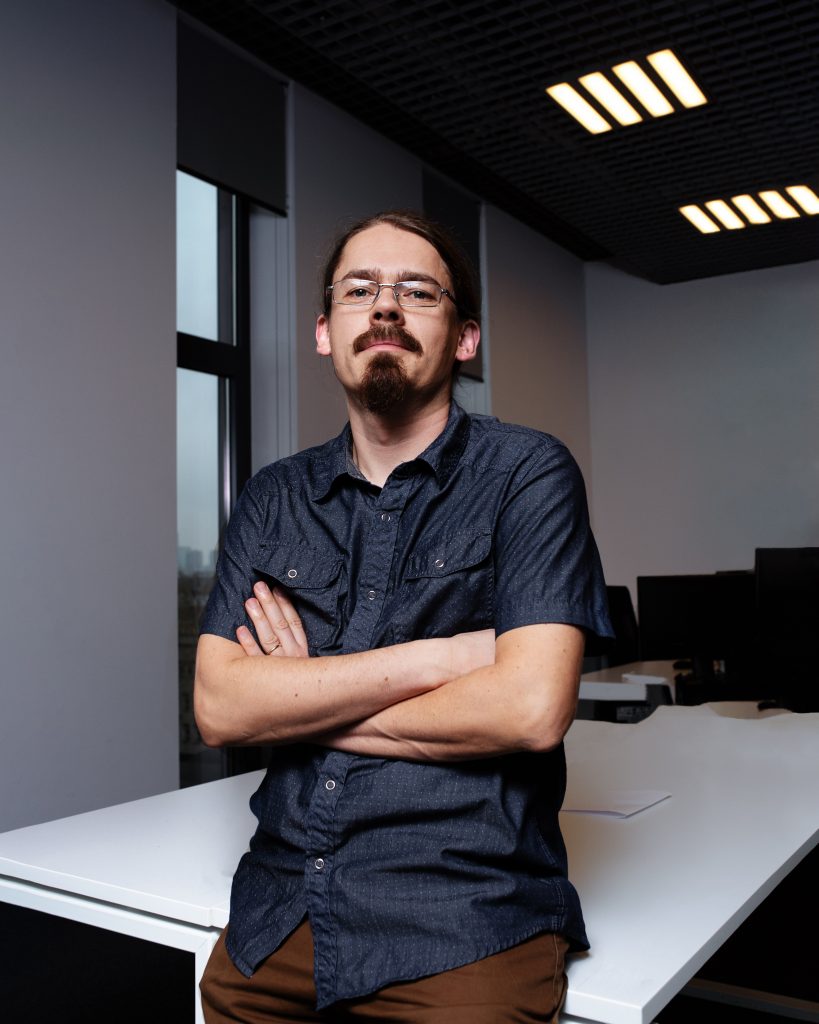
– What does your research group do?
– The group is called Silicon Photomultiplier (SiPM) for Particle Physics and Medical Physics. And my involvement with DarkSide fits perfectly with this group’s goals. DarkSide is the first experiment that uses silicon photomultipliers on such a large scale — very sensitive and modern light sensors. We are currently striving to improve our experiments with new materials so that as much light as possible reaches the photomultipliers. It is also important to increase the sensitivity of the photosensors themselves.
– How are silicon photomultipliers related to the AstroCeNT program? Tell us more about this technology.
– One of the strategic lines of work for AstroCeNT is the search for dark matter. As I said, there is a hypothesis that dark matter is a new unknown type of particle. We use liquid argon-based detectors to search for these particles. It is a gas present in the air, which is readily available and cheap, has very useful properties and is a material that is convenient to clean. To discover dark matter particles, we need to register their collisions with the nuclei of argon atoms. The nucleus moving in liquid argon causes ionization or excitation of subsequent argon atoms, which results in a short flash of light, the so-called scintillation. SiPM is used to detect this light.
Until now, classic photomultipliers made of glass were used to detect single photons (i.e. for very sensitive light detection). This technology is proven and works very well and, for example, the SNO and DEAP experiments in Canada also used it. The problem with it is that glass is relatively radioactive, and it is very difficult to clean it up to suit our ultra-sensitive experiments. So, researchers have been working on other technologies for years. Silicon photomultipliers are one solution. They can be produced in laboratories serving the electronics industry and semiconductor technologies of the highest purity. In recent years, the parameters of these devices have significantly improved.
The key issue for the sensitivity of dark matter detectors is the efficiency, i.e. how much of the photons that will appear in the experiment we will be able to register. The more the better, and we fight for every percent of the efficiency here. For example, the DEAP-3600 experiment collects roughly 20% of the light that is emitted through interactions with particles inside it. We expect that this yield will be doubled with silicon photomultipliers, so they are very attractive for science. Plus, big industry is driving research and development for this technology for general purpose applications.
– In what industries can SiPMs be used?
– There is a need for the use of SiPMs in various fields: self-driving cars, various small detectors with a photosensitive element, telecommunications, smoke detectors… So, there is a lot of pressure on the industry side for this technology to be developed.
Silicon photomultipliers can also be useful in medical physics. And here the main field of interest is PET (Positron Emission Tomography). The most important problem of PET technology is the large dose of ionizing radiation that the patient receives, which in the case of children is a significant limitation. By measuring the photon detection time much more accurately, this dose can be limited. And silicon photomultipliers are very well suited for this purpose. The AstroCeNT research group, led by Dr. Masayuki Wada, is developing the concepts of PET, based on liquid argon.
SiPMs will also be used in this device.When we equip ourselves and the group grows, we will be more active in medical physics. For now, our focus is on dark matter.
– You mentioned earlier that AstroCeNT is an attractive workplace for researchers. How are you looking for new employees?
– In parallel with the research work, AstroCeNT is now actively involved in team building. We are looking for new employees all the time. These are, as a rule, people who have physics, astronomy, engineering, or electronics education.
Our field has grown significantly in recent years. There are many research groups in the world, and the community is quite large. Usually, several hundred people participate in dark matter conferences. Nevertheless, recruiting is always a problem and it’s hard to find people with the right profile. We try to advertise widely, on portals for students, postdocs, on our website and via private channels. We are also open to cooperation with other institutions and we are actively looking for partners.
It seems to me that in Poland there is less interest in a scientific career among young people than even 10 years ago. And industry creates a lot of competition for us. But again, AstroCeNT is something completely new and much more attractive to young talented people. We implement projects that are of great importance to both science and industry. So, I believe that we will gradually build a strong team that will achieve significant success.
Photos: Dmitry Nadtocheev
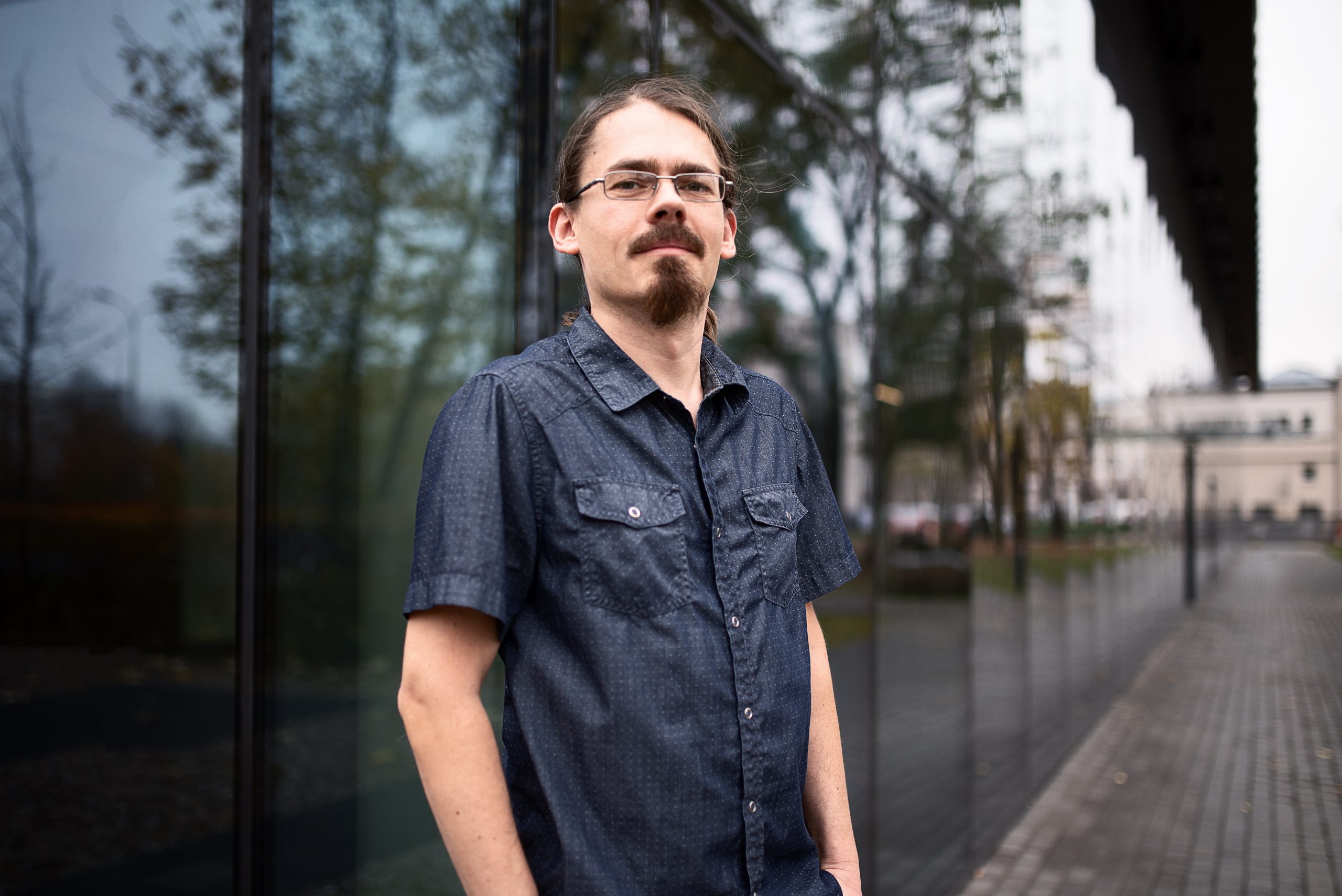